HPC User Report from T. Klein (Institute of Advanced Optical Technologies – Thermophysical Properties)
MD Simulation of Liquids with Dissolved Gases
Molecular Dynamics (MD) simulations are used to access various thermophysical properties. Good agreement with corresponding experimental data is found. The predictive capabilities and the insight in microscopic structures make MD simulations a useful tool in thermophysical property research.
Motivation and problem definition
Mixtures containing liquids with dissolved gases are relevant in numerous applications of chemical and energy engineering such as catalytic reaction technologies [1] and separation processes [2]. Accurate knowledge of the thermophysical properties of such systems is necessary for an economical process design and for modelling purposes, but still scarcely available in literature. As an alternative to experiments requiring substantial resources and effort, MD simulations can help to obtain thermophysical properties efficiently and for substances or thermodynamic conditions, where measurements are hard to realize. In a related research project funded by the German Research foundation and abbreviated by the project number DFG FR 11-1, the benefits of MD simulations are applied to get a better understanding on how especially the molecular diffusion process is affected by the characteristics of the mixture components in liquids with dissolved gases.
Methods and codes
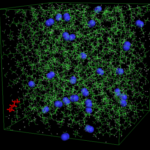
In this work, the GROMACS 5.1.2 [3] simulation software is used for performing equilibrium MD simulations of a large amount of interaction sites on the order of about 10,000, see Figure 1. To calculate, for example, densities and radial-distribution functions, corresponding tools included in the GROMACS package are used. On the other hand, for the computation of more sophisticated properties such as the interfacial tension, dynamic viscosity, Maxwell-Stefan diffusivity, and thermodynamic factor, own post-processing tools were developed. Here, mainly FORTRAN codes are used to handle the large amounts of data necessary for calculating properties from statistical fluctuations. PYTHON based software is employed for fitting and uncertainty analysis. Especially for the performance of production runs and the post-processing, the HPC cluster “Emmy” is used to parallelize the tasks on up to 6 nodes.
Results
In the current DFG-funded project, we are focusing on the prediction of mass diffusion coefficients. For binary mixtures based on n-alkanes or n-alcohols and various dissolved gases at infinite dilution, in line with theory we obtained very good agreement between the self-diffusivities from MD simulations and own experimental data for the Fick mutual diffusivity obtained by dynamic light scattering. Furthermore, first investigation of the Fick mutual diffusivity, which can be accessed by MD simulations from independent calculations of the Maxwell-Stefan diffusivity and the thermodynamic factor, showed that also the concentration dependency of the mutual diffusivity can be described well by the simulations in comparison with the experimental data. The same statement is valid for further studied properties given by the viscosity and interfacial tension of binary mixtures of liquids with dissolved gases, see Figure 2. In all cases, the resources provided by the EMMY HPC cluster helped to provide reliable data with low uncertainties, a fact which is necessary in order to judge about the capabilities of MD simulations.
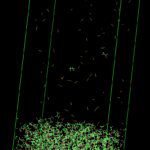
Outreach
A first paper reporting about our aforementioned findings and entitled “Mutual and Self-Diffusivities in Binary Liquid Mixtures of n-Alkanes with Dissolved Gases at Infinite Dilution” was submitted on the 22th of January 2018 to The Journal of Physical Chemistry B and is currently under review. At least two further papers connected to the current research project are planned. Furthermore, we presented results at the Diffusion Fundamentals VII 2017 in Moscow, the 21st European Conference on Thermophysical Properties 2017 in Graz, and the Thermodynamik-Kolloquium 2017 in Dresden.
References
- Maitlis, P. M.; de Klerk, A. Greener Fischer-Tropsch Processes, Wiley-VCH.; Weinheim, Germany, 2013.
- Hasib-ur-Rahman, M.; Siaj, M.; Larachi, F. Ionic Liquids for CO2 Capture-Development and Progress. Chem. Eng. Process. Process Intensif. 2010, 49 (4), 313–322.
- Berendsen, H. J. C.; van der Spoel, D.; van Drunen, R. GROMACS: A Message-Passing Parallel Molecular Dynamics Implementation. Comput. Phys. Commun. 1995, 91 (1–3), 43–56.
Researcher’s Bio and Affiliation
Tobias Klein obtained his Master of Science (M.Sc.) degree in Energy Technology at the Friedrich-Alexander-Universität Erlangen-Nürnberg (FAU). Since April 2016, he is working a doctoral candidate in the Institute of Advanced Optical Technologies – Thermophysical Properties (AOT-TP) under supervision of Prof. Dr.-Ing. habil. Andreas P. Fröba.